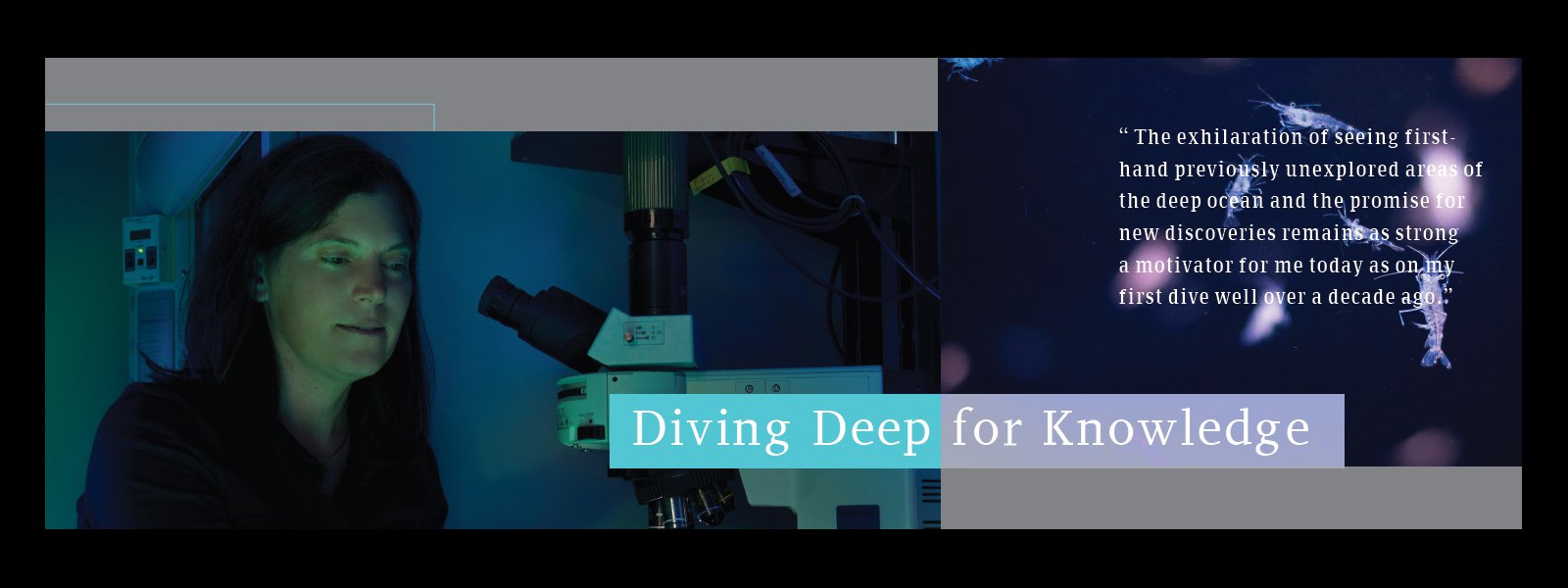
SPARK | The Daring Journey | Victoria Orphan | NOMIS Article
I'm an explorer at heart. As a child, I was always drawn to the ocean and the mysterious animal life below its surface. As an adult, I'm still captivated by the ocean's vast and powerful life-sustaining role on Earth, but I've turned my attention away from the charismatic tropical fish, coral reefs, and marine mammals that captured my imagination in my youth to a vibrant ocean world of microbes that often goes unnoticed. This world is invisible to our unaided eye — teeming with single-celled bacteria, archaea, protists, and viruses, the smallest life forms and among the most difficult to study. They number among the million trillion trillions in ocean waters and deep sediments, far surpassing the total number of stars in our solar system.
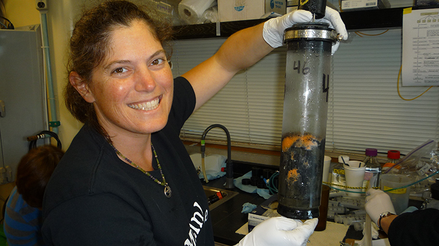
Researchers in the relatively young field known as geobiology are just beginning to understand the intricacies of this complex microbial world and how it has changed over the course of Earth's history. However, what is clear is that the collective metabolic activity of microorganisms in terrestrial and marine environments continues to fundamentally shape the global biogeochemical cycling of carbon and nutrients. Microbes regulate greenhouse gases in our atmosphere and play a foundational role in many important ecosystem services that sustain the diversity and productivity of animals and plants. Quantifying the metabolic roles and interactions of microorganisms in the environment has been far from straightforward. The vast majority of microorganisms in the wild have resisted domestication, with over 99 percent of the microbial species observed in nature not cultivable in the laboratory.
The profound ecological importance of microorganisms in the global geochemical stage continues to inspire my research, but I am also motivated by the challenge of figuring out how to study and experiment on microbial communities in nature — especially those living in the deep ocean. As a geobiologist, the ability to work directly in the environment has been invaluable. The exhilaration of seeing firsthand previously unexplored areas of the deep ocean and the promise for new discoveries remains as strong a motivator for me today as on my first dive well over a decade ago.
As a graduate student in a molecular microbial ecology lab, I had the opportunity to collaborate closely with organic and isotope geochemists, petroleum geologists and earth scientists. From this experience, I gained a deep appreciation for the benefits and challenges of cross-disciplinary collaborations. Perhaps the most formative of these were my early interactions with organic geochemist Kai Hinrichs and earth scientist Christopher (Chris) House, who introduced me to the field of stable isotope geochemistry. Kai shared my passion for methane-eating microbes, and Chris, then a new professor in the geosciences at Penn State University, had been applying stable isotope techniques to assess microbial carbon metabolism — in this case he was measuring the 13C values of billion-year-old microfossils hypothesized to be ancient cyanobacteria using a specialized technique known as secondary-ion mass spectrometry (SIMS). At the time, SIMS was used in the geosciences and material sciences, but its application in microbial ecology to study the metabolism and interactions of modern environmental microorganisms had not yet been attempted. This uncharted territory presented an exciting opportunity to merge our collective expertise to see if we could directly combine molecular RNA-based fluorescence imaging (known as FISH) — which I had been using to identify methane-oxidizing archaea based on their unique ribosomal RNA sequence in environmental samples — with SIMS to analyze the stable carbon isotope composition of the FISH-stained archaeal cells.
While this experiment appeared relatively straightforward on paper, learning each other's scientific vocabulary and discovering the inherent limitations associated with each of our respective methodological approaches was a challenge. I still remember my dismay after discussing the details of the SIMS instrument with Chris over the phone, having realized that many components of the standard FISH protocol I had been using needed to be revised to make it compatible with SIMS. How would we create a roadmap of our FISH samples to find micron-sized target cells on a 25 mm SIMS wafer without the aid of fluorescence and at low magnification on the SIMS instrument? Would the mapped cells stay adhered to the solid support under the ultrahigh vacuum of the SIMS instrument? There were a lot of unknowns and methodological uncertainties to resolve. But our early communication and iterative method revisions paid off when we successfully made the first-ever isotopic analysis of these uncultured archaeal cells, showing that, as hypothesized, they had a carbon-13 signature that was consistent with methane consumption. Experiencing the raw excitement of this collaborative discovery and overcoming the setbacks in the scientific process solidified my love of science and I still carry the lessons learned into my research today.
As little as we know about the microbes themselves, we know even less about the viruses that infect them and how they influence the flow of carbon and major nutrients in the deep sea. I'm looking forward to this new chapter to fill in the important knowledge gaps relating to the transfer of carbon and nitrogen through these submicron-sized microbial predators.
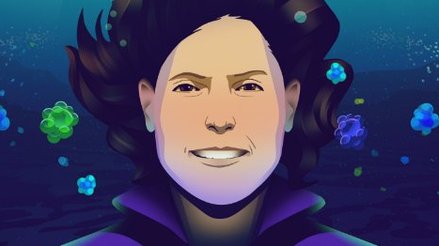